In synchronization with their counterparts in America, Europe, Australia and India, a team of Chinese astronomers announced on June 29 their successful detection of the first evidence for the gravitational wave (GW) background in the nanohertz waveband (10-9 Hz, nHz), a range of extremely low frequencies. They identified the signature fingerprint of this humming background from the massive data accumulated in their observations starting in April 2019, with the aid from an array of 57 millisecond pulsars scattered across the Milky Way.
They summarized their results in a paper published online in the journal Research in Astronomy and Astrophysics, followed by a series of papers delving into details, all using the data obtained by FAST monitoring the widely spaced pulsars.
Their counterparts in Europe, America and Australia have been working decade-long to hear the whispers of nanohertz GW background (GWB), as a result from the superposition of countless overlaid GWs created by the universe. Interestingly, despite the fact that they conducted the observations independently using different combination of pulsars, their findings agree with each other very well, all recognizing the same correlation pattern between the pulsars as predicted a century ago by Einstein’s theory of general relativity. For astronomers, this is both reassuring and exciting: they previously expected some disagreement in the results, considering different pulsars are located in different relative distances from the sources of nHz GWs. The somewhat surprising isotropy, which indicates the existence of an isotropic stochastic GWB, throws more mysteries over the unidentified sources.
The Background Humming of the Universe
GWs, often compared to ripples of the universe, are disturbances of spacetime itself transmitting throughout the cosmos. Albert Einstein predicted in 1916 the existence of this imperceptible vibration based on his theory of general relativity. According to the theory, gravity is actually the distortion of spacetime itself in response to mass, and the accelerated movement of massive bodies in turn can ruffle the adjacent spacetime to create vibrations. Such vibrations propagate in the universe just as how ripples spread through a water body, and hence produce gravitational waves. His prediction was first proven in the 1980s by R. Hulse and J. Taylor, who won the Nobel Prize for this; and later proven by solid observational evidence in September 2015, when the Laser Interferometer Gravitational Wave Observatory (LIGO) directly observed the GW “siren” produced by the spiral closing-up and merger of two stellar black holes. The two black holes, respectively of 36 and 29 solar masses, bellowed out this “siren” from 1.3 billion lightyears afar, raised its tones from dozens to hundreds hertzs, about 1011 times higher than the newly found nHz GWB.
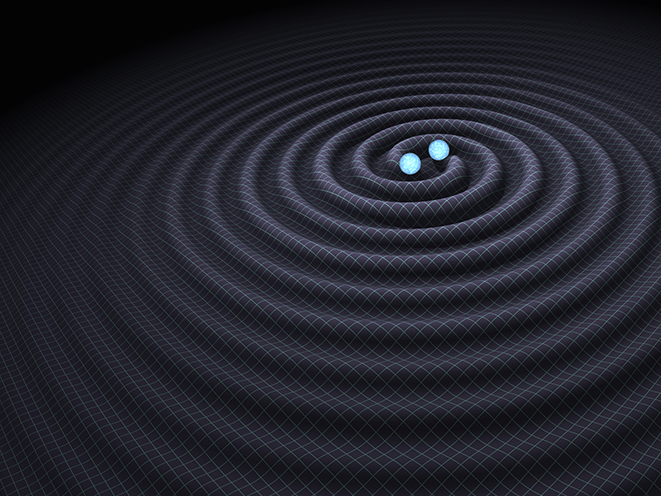
This illustration shows the merger of two black holes and the gravitational waves that ripple outward as the black holes spiral toward each other. In reality, the area near the black holes would appear highly warped, and the gravitational waves would be difficult to see directly. (Credit: R. Hurt/Caltech-IPAC)
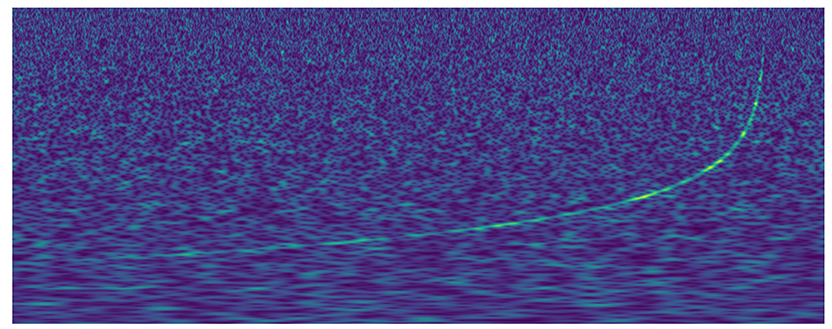
The spectrogram, combining the signals from the two arms of LIGO, the Hanford and Livingston detectors, shows the characteristics of the “sweeping chirp” of a gravitational wave detected on October 16, 2017. The gravitational wave was given off by the closing-up and collision between two neutron stars. When the two came closer to each other, they circled faster, and hence produced higher frequency gravitational waves, as shown by the greenish line “sweeping” upwards. (Credit: LSC/Alex Nitz)
Unlike what was first detected by LIGO, the wavelengths of nHz GWs can go as long as a dozen lightyears, undulating at periods of years in length. This weak, deep voice might inform us of interactions between colossal celestial bodies like supermassive black holes and large galaxies, as theoretical simulations predict that GWs produced by merger events between thus massive bodies would likely fall in the nHz waveband.
But the observation of nHz GWs is unimaginably difficult.
Gravity itself is a weak force; GWs as the aftermath of its agitation, are imperceptibly dim and weak, too. They are so weak that their detection requires colossal antennae. To detect GWs in the high-frequency band, LIGO adopts an antenna with two arms each spanning four kilometers. Unfortunately, if the same observational method applied, the lower the frequency, the bigger the antenna would have to be. To detect the faint signals of nHz GWs, human beings would need to get a gargantuan instrument as big as the Milky Way.
Ground-based GW observatories can only detect GWs in the high-frequency band. Shown here is the data obtained by LIGO on January 4, 2017 indicating a gravitational wave given off by a colliding binary of black holes. The frequency raises from dozens to hundreds. (Credit: Caltech/LIGO/MIT)
Gravitational waves in different wavebands and their sources. (Credit: NASA/J. I. Thorpe)
How can humanity build such a gigantic instrument anyway?? How come a telescope as “small” as FAST, with an aperture of 500 meters only, has actually heard such incredibly faint hums?
Antenna “as Big as the Milky Way”
“Actually, the real detector for nHz GWs is not FAST or other participating telescopes, but pulsars in the universe. They form a huge antenna for GWs, one as big as the Milky Way,” explains Dr. Lee Kejia, a researcher at the National Astronomical Observatories, Chinese Academy of Sciences (NAOC), the institution in charge of FAST.
Lee works as the Principal Scientist for the project in search of nHz GWs, the Chinese Pulsar Timing Array (CPTA), which is a big collaboration joining an array of astronomical observatories under the architecture of the Chinese Academy of Sciences (CAS), as well as top universities of the country. “We only use large telescopes like FAST to read pulsar signals, in order to check any deviation in their time of arrival (TOA), hence to know how the spacetime has been stretched or squeezed by possible GWs that happen to pass by,” he says.
Scientists go a smart way and harness a type of magical celestial bodies in the universe, pulsars. These “pulsating stars” are generally understood as remnants from supernovae, which collapsed towards their own cores in the wake of the explosions, and condensed into compact neutron stars. They keep radiating electromagnetic beams from their poles, as a result from the unknown radical mechanisms continuing inside, and spin at very fast, yet highly stable speeds. Their rotation can stay so regular that the accumulated error in time consumption might not go beyond even one second over an extremely long time – for some millisecond pulsars, it takes them over one hundred million years to make that much “mistake” in spinning speeds. This striking accuracy makes them ultra-precise clocks in the universe. In other words, by observing and counting their pulses, we can know the time.
If these accurate “clocks” themselves drift back-and-forth and wobble up-and-down following the ripples of GWs, we will know from the delayed or early arrival of the pulses, and the changes in their inclination, right? Any deviation in TOA could mean some disturbance in spacetime.
Due to the offset between the directions of pole and rotation, the electromagnetic radiation beams of pulsars only arrive at the earth at intervals: spinning fast, only when their polar emission beams sweep across our planet can we hear the “pulses”. Therefore, any change in the incoming elevation and frequency will mean something.
Coming unfailingly at accurately constant intervals, these pulses can be used as a highly reliable calibration for spatial distance and movement. When we know the exact rotational speeds of every pulsar in an array, we will be able to use the whole set of “clocks” as a refined grid to measure the slightest distortion, if any, in the surrounding spacetime.
An artist’s interpretation of a pulsar. The basics of a pulsar are color-coded. In white is the neutron star. Its powerful magnetic field is shown in blue. The north and south poles of that magnetic field, and the directions from which the pulsar’s beams shoot, are in yellow. And in red is the axis of rotation of the star. That axis is offset from the beam, which is why when the star spins, the beam sweeps past us. (Credit: B. Saxton, NRAO/AUI/NSF)
More importantly, without the influences from the background of GWs and noises, these widely spaced clocks, each separated by hundreds to thousands of lightyears, would “tick” totally independently from each other; while in the presence of GWs, they would tick in an orchestrated way fitting a certain pattern. In other words, the anomalies in the ticking rate of this set of ultra-precise cosmic clocks, should correlate with each other in some certain pattern, responding to the same disturbances. This very pattern, was also predicted by Einstein’s theory of general relativity as the fingerprint of the humming GWB.
Listening to the Humming Background
Therefore, as long as we can accurately measure these anomalies, we will be able to tell whether a GW has come. In fact, based on the understanding that in the universe, lots of GWs have risen since the Big Bang, or the birth of the universe, we should expect the outcome of the superposition of many GWs – the GWB, instead of a single chain of GW.
Still, to dissect the nanohertz part of the GWB by real astronomical observation is challenging. Before we can really make it, at least two conditions have to be met. First, we know the rotation of these pulsars well enough; second, we can measure the anomalies at an accuracy good enough for this extremely small disturbance.
Thanks to the long-term collective efforts of the global astronomy community, human beings have known very well about the rotational properties of a number of pulsars. Monitoring an array of such well-known pulsars, which rotate very regularly, astronomers can track the deviations in TOA caused by the propagation of nHz GWs across the spacetime, if they do come. Recording the TOA errors at different localizations, a pattern of correlation between pairs of pulsars can be reconstructed to tell whether the culprit of the disturbance is the GWB or merely noise, and such a benchmark pattern was already established by Hellings and Downs (HD) in 1983 as a function. If data obtained from the pulsar array fits the signature correlation curve of this function as described by them (termed Hellings-Downs correlation, or HD-correlation), astronomers will know the anomalies are caused by the GWB.
The accuracy of observation also matters – after all, GWs themselves are already dim and faint enough to drop out of the measuring range of most observatories, let alone the fluctuations of TOA errors caused by their propagation. Aside from the observational sensitivity and accuracy, long-term work is a must to improve the reliability of the data, which is in proportion to the accumulative timespan of observation. Therefore, for the time being, the only possible way to directly detect nHz GWB lies in the long-term, systematic TOA observation of pulsars. Given the extremely low frequencies of the target GWB, it can take decades to know whether the result is reliable enough.
It is doomed to be a long, arduous expedition, somewhat audacious.
Consortium to Chase the Fingerprint of nHz GWB
This did not scare the astronomers away, however. To solve the problem, they turn to large spherical radio telescopes to time the pulsars, tracking the subtle anomalies in TOA across the galaxy-size array. They endeavor to search for the HD-correlation, the footprint of GWB, out of the massive volume of data.
An international observational campaign has hence risen in the mid-2000s to explore this special type of signals. Some regional Pulsar Timing Arrays (PTAs) came into being as forerunner detectors, namely the European Pulsar Timing Array (EPTA) in Europe, North American Nanohertz Observatory for Gravitational Waves (NANOGrav) in America, and the Parkes Pulsar Timing Array (PPTA) centered around the Murriyang Parkes Radio Telescope in Australia. To coordinate the observations and data shared between them, the three regional PTAs set up a global consortium for GW detectors, namely the International Pulsar Timing Array (IPTA), in 2008. The later years saw three more regional PTAs join the party, including the Chinese Pulsar Timing Array (CPTA) and the Indian Pulsar Timing Array (InPTA) in Asia, and the South Africa Pulsar Timing Array (SAPTA) in Africa. Among them, InPTA has joined IPTA as a formal member, and SAPTA and CPTA are currently observers of the organization.
The main telescope of CPTA is the newly built FAST. It boasts an aperture of 500 meter, the biggest of the world, but only came into test operation in April 2019, while by the time the forerunner TPAs, EPTA, NanoGrav and PPTA, had been staring at the pulsars for over a decade.
Fortunately, the telescope’s outstanding sensitivity, accuracy and ability to monitor multiple objects at once, help them catch up.
HD-correlation observed: The CPTA team measured the correlation coefficients (y-axis) between different pairs of pulsars, and obtained them as a function of the pulsar-pair separation angle (x-axis). The signature HD curve is clearly seen as depicted by the solid red curves. Graphs from left to right correspond to GWs of different frequencies: f1 = 9.34 nHz, f1.5 =14 nHz, and f2=18.7 nHz, respectively. (Credit: CPTA)
The CPTA team chose an array of 57 pulsars whose spinning periods are as short as in milliseconds. The team systematically time this array pulsars from April 2019 on, when FAST was still doing test observations. Now, with data from only 41 months’ observations, the team identified the signature HD-correlation curves corresponding to different frequencies in the nanohertz band. For the GWB component of 14 nHz, they found an HD-correlation at a statistic significance of 4.6σ, corresponding to a P-value of 4×10-6. This means, the chances for a mistakenly caught culprit (say, noise instead of GWB) is extremely slim, down to only 0.000004. The statistical significance varies across frequencies.
“We saw the deviation in TOA caused by the ripples of spacetime,” says Prof. CHANG Jin, Director of NAOC and a Member of the Chinese Academy of Sciences. “This demonstrated the existence of nHz GWB.”
Ubiquitous, Stochastic Background
What has produced the detected nHz GWB is still pending for examination and interpretation. Scientists emphasize that further observations are needed to understand the data and localize the sources.
Gravitational waves serve as a new messenger for cosmic exploration, as it offers us the possibility to look into the immemorial time when the universe was just born, far before the first beam of visible light made its debut. Particularly the primordial gravitational waves produced by the Big Bang, may help us understand the infantile Universe. (Image: NAOJ)
Essentially, cosmologists understand that lots of scenarios can produce nHz GWs. Examples include mergers of supermassive blackhole binaries and interactions between large galaxies, as mentioned earlier. More interestingly, the Big Bang occurring 13.8 billion years ago could also have produced a cascade of GWs, and the inflationary expansion of space that immediately followed could have amplified their signals. If the low-frequency residual from these GWs can be detected, we will have a new channel to look into the infantile universe, where no optical signal is available to bring us any information.
The isotropic characteristic of the detected nHz GWB is another intriguing part. What could this mean? Seems that GWs of this low frequency are ubiquitous, resting as a stochastic background for the visible astronomical events on the front stage. Could it be something like and better than the cosmic background (CMB) radiation, bringing us the message about the early days of the cosmos?
“The newly detected nanohertz GWs open a new window for us on the universe, and promise many important discoveries in physics. From this new window, we might be able to look into the supermassive celestial bodies, like supermassive black holes; we could be able to understand the formation, evolution and merger of galaxies; and we might even be able to understand the early structure of the universe,” comments Prof. CHANG Jin, NAOC Director.
Gravitational waves serve as a new messenger for cosmic exploration, as it offers us the possibility to look into the immemorial time when the universe was just born, far before the first beam of visible light made its debut. Particularly the primordial gravitational waves produced by the Big Bang, may help us understand the infantile Universe. (Image: NAOJ)
Now the observation is still going on. The CPTA team expects an improved statistical significance with the further accumulation of data.
“In the future, we expect that data with a longer span will enable us to go lower in frequency and therefore measure the spectral index with better accuracy,” the authors indicate in their summary paper.
Also, the team expects a further increase in detection sensitivity by combining IPTA and CPTA data in the future. CPTA’s second data release, scheduled for 2026, is also expected to deliver better accuracy in GWB parameter inference.
“We expect that the future IPTA data analysis and the next CPTA data release will be more sensitive to the nHz GWB, which could verify the current results,” say the authors in their paper.
References
Hellings, R. W., & Downs, G. S. 1983, Upper Limits on the Isotropic Gravitational Radiation Background from Pulsar Timing Analysis. The Astrophysical Journal, 265: L39–L42.
Jenet, F. A., Hobbs, G. B., Lee, K. J., & Manchester, R. N. 2005, Detecting the Stochastic Gravitational Wave Background Using Pulsar Timing. The Astrophysical Journal, 625: L123–L126.
Manchester, R. N. 2008, The Parkes Pulsar Timing Array Project, in 40 YEARS OF PULSARS: Millisecond Pulsars, Magnetars and More. AIP Conference Proceedings, 983: 584–592 (2008).
Manchester, R. N. & IPTA 2013, The International Pulsar Timing Array. Classical and Quantum Gravity, 30, 224010. DOI: 10.1088/0264-9381/30/22/224010
Sesana, A. 2013, Systematic investigation of the expected gravitational wave signal from supermassive black hole binaries in the pulsar timing band. Monthly Notices of the Royal Astronomical Society, Vol. 433, p. L1-L5. DOI: 10.1093/mnrasl/slt034
Xu, H. et al. 2023, Searching for the Nano-Hertz Stochastic Gravitational Wave Background with the Chinese Pulsar Timing Array Data Release I. Research in Astronomy and Astrophysics. 23, 075024. DOI:10.1088/1674-4527/acdfa5